Organic chemistry is a field where many areas are focused on carbon atoms as the basic structural component of each compound from that group. Most organic compounds also contain in their structure hydrogen and oxygen atoms, as well as nitrogen, phosphorus, sulphur and chlorine atoms. However, the most important molecule is carbon, given its special capability to produce bonds. For this reason, there exist such structurally simple organic compounds as single-carbon methane, but also DNA, which includes tens of billions of atoms.
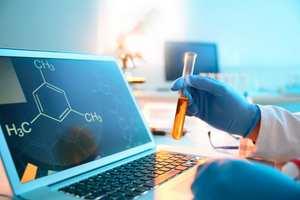
History of the theory of carbon bonds in organic compounds
As early as in 1858, two scientists, August Kekule and Archibald Couper, issued independent works in which they have claimed that the carbon atom in all organic compounds is capable to attach four substituents. It was then defined that carbon had four centres of affinity with other units, which meant in practice that four-valence carbon atoms are capable to produce four chemical bonds, which leads to the formation of stable compounds.
August Kekule has also claimed that carbon atoms can link with one another to produce longer carbon chains. The next step were theories on the possible existence of multiple bonds. Alexander Crum Brown proposed the existence of a double C=C bond in ethylene, while Emil Erlenmeyer – the existence of a triple C≡C bond in the acetylene molecule. In 1865, Kekule made another contribution by developing the concept of carbon atoms linking not only in a simple way but also with bending and closing to form rings. In 1874, Jacobus vant’t Hoff and Joseph Le Bel introduced another dimension to the chemistry of molecules by assuming that carbon bonds existing in space are not oriented in a chaotic manner but have a specified spatial arrangement. In this way, we have reached the presently existing model of the quadrivalent carbon atom, whose bonds in space form a tetrahedron, and its corners are arranged not only on a flat line but also in front of and behind the plane of the observer’s line.
Why are chemical bonds formed?
Atoms bond with one another to make the produced molecule as stable as possible. Such a form is more durable and has a lower energy than individual atoms separately. Upon the formation of a chemical bond, the energy of the system is released and reduced. The analysis of the formation of such bonds is also based on the information about the state of their electron valence shell. The fact is that the highest stability is shown by those elements which have an octet in that shell, while the elements which do not have an octet in a free state are prone to adopting the electron configuration of a noble gas. Depending on the group, the path to that state may require the loss of an electron, for example. The amount of energy necessary to perform such an operation is defined by the ionisation energy (Ej).
Ionic bonds
The metals situated on the left side of the periodic table tend to produce positive ions (cations) by giving out their electrons. On the contrary, halogens and other reactive non-metals attract additional electrons, thus producing negative ions (anions). The key element that affects the behaviour of atoms relative to electrons is their electron affinity (Epe), which is specific to each of the atoms analysed. An electron attaching to most elements causes the release of energy, so most Epe values are negative. The ionic bond is the simplest case for analysis – it exists between a metal with a low Ej value and a non-metal with a high absolute Epe value.
The atomic bond
The elements located in the middle of the periodic table cannot form ionic bonds due to their electron configuration. Carbon, the element most important for organic chemistry, if we consider the simplest structure of methane (CH4), has the following configuration: 1s12s22p2, so it would be very difficult for it to accept or give up electrons to achieve the configuration of a noble gas. The atoms of this type act in a different way, by sharing their electrons with other atoms.
Such a scheme was first proposed by Gilbert N. Lewis in 1916. The bond was called the covalent bond, and the set of atoms linked to it was called the molecule. The method of presenting the notation of such bonds is based on Lewis’s structural formulas, in which the valence electrons are represented by dots. The highest molecular stability is achieved when the configuration of the atom reaches the electron configuration of a noble gas and has its valence s- and p-orbitals filled. The number of atomic bonds possible to be formed depends on the number of the atom’s valence electrons. If the atom contains one, two or three valence electrons, then this is the number of bonds it can produce. If the atom has four or more valence electrons, it produces as many bonds as are sufficient to fill the s- and p-levels of their shells, until an octet is reached.
The theory of valence bonds
It assumes that an atom-type chemical bond is formed when the atoms approach each other at such a distance that their individually filled orbitals overlap. The electrons paired in that way are attracted to the nuclei of both atoms, while linking with each other. The strength of such a bond largely depends on the degree the orbitals overlap: the bigger the overlap, the stronger the bond. This leads us to the next point of the theory: the orientation of the bonds in the case of the overlapping of orbitals other than s. If there is an interaction, for example between a 2p- and 1s-orbital, it is developed along the axis of the directional p-orbital. Based on the shape of the overlapping orbitals, we can also obtain the cross section of the bond. To give an example: if two hydrogen atoms share electrons from the s-orbital shaped as a sphere, the cross section of that bond will also be a circle, and the symmetry of the H-H bond will be cylindrical. A bond formed by a frontal overlap of orbitals along the line between the nuclei is a sigma (σ) bond, which is most common. Another one is the pi (π) bond, being a result of the overlap of individually filled 2p-orbitals. It prevents rotation, for example around the C-C bond. It is weaker than the sigma-bond and easier to break, which makes it more reactive.
The most important assumptions of the theory of valence bonds are the following:
- Atomic bonds can be formed thanks to the overlap of molecular orbitals that have one electron with an opposite spin.
- Each atom taking part in bond formation keeps its atomic orbitals, while the electron pair located in the overlapped orbitals is shared.
- The more the orbitals overlap, the stronger the chemical bond.
Terms such as bond strength or bond length are specific to each atomic bond.
Strength – defines the amount of energy that must be supplied to the system in order to break the molecule into atoms. It can be calculated by comparing the energy of the stable product to the energy of the substrate. For example, if the connection of hydrogen atoms causes the molecule to release 436 kJ/mol, the product’s energy will be lower by that value, and this will be the strength of the bond. In order to break it, we would have to supply at least that amount of energy.
The length of a bond refers to the optimal distance determined between the nuclei. If they are too close, they will push each other away due to their positive charge. If they are too far from each other, they will be unable to share their bonding electrons.
The theory of molecular orbitals
It assumes the definition of atomic bonds as mathematical combinations of wave functions that form molecular orbitals. They are considered as components of the whole molecule, not of a particular atom. Therefore, a molecular orbital defines the part in the molecule’s space where the electrons are most likely to be present.
As in the case of atomic orbitals, the characteristic values include size, shape and energy. Unlike them, molecular orbitals may interact in two different ways: additively or subtractively. Taking the hydrogen molecule as an example, if the orbitals link additively, their shape will resemble an egg. Subtractive linking will cause mutual subtraction of these orbitals, and the space between the nuclei will only include a wave function node, which results in a low density of the electron cloud and the lack of filling. In the case of additive linking, the orbital’s energy is lower than that of individual 1s-orbitals of a hydrogen atom and constitutes a bonding orbital. The energy of an orbital formed by subtractive combination is higher than the energy of individual atomic orbitals, and such an orbital is referred to as an antibonding orbital. This is due to the fact that the electrons present in such an orbital cannot be situated in the node space and, in consequence, they are unable to produce bonds. The basic assumptions of the theory of molecular orbitals include the following:
- Molecular orbitals are the same part in molecules as atomic orbitals in atoms. Since they are possible to describe, we can present the spacial part in the molecule where electrons are most likely to be present. Each molecular orbital has its specific size, shape and energy level.
- Molecular orbitals are formed by the conversion of atomic orbitals. Their number is equal to the number of the present atomic orbitals that produced the combinations.
- Depending on the energy of a molecular orbital in relation to atomic orbitals, they can be bonding or non-bonding. If the energy of a formed molecular orbital is lower, they are bonding orbitals; if it is higher, they are non-bonding orbitals.