Proteins are large biomolecules which are the basic structural components of all living organisms. They are macromolecular compounds containing α-amino acid residues and amide bonds, otherwise known as peptide bonds. The molecular weight of proteins exceeds 10,000 daltons (Da). The peptides of lower molecular weights constitute a separate group known as oligopeptides. Common proteins are made of up to twenty amino acids, all of which (except glycine) have stereochemistry similar to that of L-sugars. Depending on the composition, these chemical molecules can be classified into two groups. First of those includes simple proteins, i.e. proteins that hydrolyse into amino acids only. This group includes e.g. serum albumin. The second group includes complex proteins that hydrolyse into products containing amino acids and other compounds, such as carbohydrates, fats and nucleic acids.
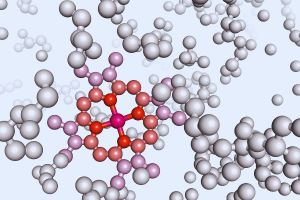
The chemical composition of proteins
The basic chemical parameter defining a given group of compounds is their chemical composition. Using elemental analysis, it was determined that the structure of each representative of the protein group contains carbon (50-55%), hydrogen (6-7%), oxygen (20-23%), nitrogen (12-19 %), and smaller amounts of phosphorus (0-6%) and sulphur (0.2-3%).
Classification of proteins due to their three-dimensional form
Proteins are also classified as fibrillar and globular, referring directly to their three-dimensional form. Fibrillar proteins are made of polypeptide chains forming long fibres. They are resistant to and insoluble in water, and so they serve as a building material for structural tissues, e.g. tendons, hooves, nails, connective tissues of blood vessels and muscles. The most common representatives of this group are collagen and creatine, and also fibrinogen, elastin and myosin. Globular or glomerular proteins are molecules in the form of folded compacts, resembling a sphere. These proteins are usually well soluble in water and migrate freely within the cell. This type of structure is typical for most of known enzymes, haemoglobin, immunoglobulins, insulin and ribonuclease. The use of globular proteins includes oxygen transport processes, immune reactions and hormonal and enzymatic regulations, including glucose metabolism and RNA synthesis.
Amphoteric properties of proteins
A relatively large amount of ionizing amino acid residues present in globular proteins gives them the property to act in solutions both like acids and alkalis. Their properties are environment-dependent. In acidic solutions, due to vast amounts of hydrogen ions, the dissociation of acidic groups reverses, making the protein molecule a cation. In the opposite situation, however, the molecule is an anion – in an alkaline environment alkalic groups lose their electric charge. Due to this dual nature, proteins can dissociate in two ways – in an acidic and alkaline way.The dissociation degree and number of charges depend directly on the pH and the type of amino acids present in the structure. There is also the isoelectric point, i.e. such environmental pH in which there is an equal amount of positive and negative charges, and the protein molecule becomes a zwitterion. This value is characteristic for individual proteins, andmakes it possible to separate them. At the isoelectric point:
- the smallest value of electric charge and the lowest conductivity are observed,
- proteins show the lowest mobility, and thus most of them precipitate or turn into a sol/gel,
- such properties as: viscosity, swelling capacity, solubility, osmotic pressure are the lowest.
The structure of proteins
This compound group is characterized by extremely large structures at four different levels. Simply put, a protein is a sequence of binded amino acids. This is the most basic level of their arrangement – the primary structure.The secondary structure is a certain regular arrangement, resulting from the bending of the polypeptide core, and thus from its three-dimensional structure. Further, the tertiary structure involves the classification based on the folding of the molecule into its final shape. The quaternary structure describes the formation of larger aggregates by a given protein molecule.
Enzymes
Enzymes are a group of large proteins acting as catalysts in many biological reactions. They differ from laboratory-used chemical catalysts due to their specific action. Typically, one enzyme is able to catalyse only one reaction of only one compound, called the substrate. For example, the human GI system contains amylase, which catalyses the hydrolysis of starch to glucose only, and does not catalyse the hydrolysis of cellulose or other polysaccharides. There are also other enzymes, such as papain, acting on a whole group of substrates, in this case catalysing the hydrolysis of many types of peptide bonds. Similarly to chemical catalysts, enzymes do not disturb the constant equilibrium of a reaction, but only lower the activation energy, which results in the acceleration of the process.
Protein denaturation
Globular proteins have a tertiary structure that is held in balance by weak intramolecular interactions. It is very easy to disrupt it, e.g. by even a minor change in temperature or pH. This, in turn, results in protein denaturation. However, these conditions are so minimally invasive that covalent bonds are not broken. Thanks to this, the primary polypeptide structure does not change. However, each other structure does, which leads to its unfolding from a spherical shape and transforming it into a chaotic bundle. Nevertheless, there are other factors that cause protein denaturation, such as UV rays, strong shaking, high pressure, and a number of chemical factors, including strong acids and heavy metal salts. The denaturation of proteins causes significant changes in their physical and chemical properties. Their solubility decreases drastically, which can be observed, e.g. when cooking eggs: albumins unfold and coagulate into an insoluble white mass in the form of curd protein. Due to denaturation, most enzymes lose their biological activity, because the key tertiary structure is destroyed. Post denaturation, the activity of the exposed chemical groups increases, the rotation angle of the plane of polarized light increases, and the susceptibility to proteolytic enzymes increases. Usually, the process of denaturation is irreversible, but spontaneous renaturations occur at the initial stage of molecule unfolding. Enzymes then also regaintheir previously lost biological activity. Based on this observation it can be concluded that their tertiary structure completely returns to its stable form.