It is a branch of physical chemistry that deals with processes in terms of the probability, energy effects, and states of equilibrium. Thermodynamics is an empirical science that requires experiments, observations, developing theories that explain the course of specific reactions, and predicting the outcomes of further experiments.
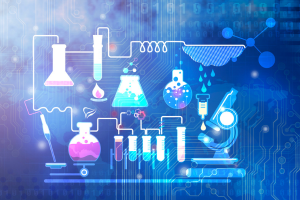
System
To discuss chemical thermodynamics, it is necessary to clearly define the system and its surroundings. A system is a portion of matter with specific physical and chemical properties, which is separated from its surroundings by walls. The surroundings is the rest of the universe that is outside the system. Examples of a system include a beaker filled with water, a cylinder with a gas, or a biological cell. We distinguish several terms that define systems:
- An open system means that matter and energy can be exchanged with the system’s surroundings, for example an open reactor.
- A closed system means that matter cannot be exchanged, for example a closed flask. However, the exchange of energy is possible. Closed systems can be adiabatic (if they do not exchange mass nor heat but they exchange other forms of energy; for example: work) or isothermal (if they do not exchange mass but they exchange all forms of energy).
- An isolated system means there is neither matter nor energy exchanged with the surroundings, for example an insulated and closed vacuum flask.
State parameters
Each system has its defining physical quantities called variables or parameters, which can be:
- extensive, where they are affected by the amount of substance present in the system, for example volume or the number of moles;
- intensive, where they are independent of the amount of substance in the system, for example molar fraction, specific volume, or temperature.
The mentioned parameters determine the basic values of thermodynamic functions, specific to systems and processes. These include: internal energy (U), enthalpy (H), entropy (S), free energy (F), free enthalpy (G) and their derivatives. In the case of a one-component system containing 1 mole of a substance, these values refer to molar thermodynamic functions designated with the additional letter “m” in the subscript. In thermodynamics, one must always use proper units; for temperature we use the Kelvin scale, where 0°C = 273,15 K, while for pressure it is 1 pascal.
Reversibility of a process
During physicochemical processes, the system’s initial parameters are changed. Once the process is finished, they can be restored if a reverse reaction occurs. Thus the system returns to the initial values, i.e. the process is reversible. However, if the amount of mass, heat or work exchanged with the surroundings during the reaction do not balance each other out when the reaction ends, then the process is considered irreversible. It can only proceed in one direction, until at least one substrate is exhausted. Processes can also be spontaneous (where they occur at the expense of the system’s energy) or induced (where energy must be acquired from the surroundings). If there are no force or energy gradients between the system and the surroundings, and the system parameters remain unchanged, the system is deemed to be in the state of equilibrium.
Phases
Systems can not only include one or more components but also one or more phases. In a system, a phase refers to its part throughout which physical and chemical properties are uniform. There are visible interfaces in multi-phase systems. The simplest example of different phases is water in three different states: liquid, gaseous and solid. When describing phases, it is necessary to specify not only the temperature and pressure but also their qualitative and quantitative compositions.
Internal energy (U) of a system
It is the sum of energies of the matter contained in a system, which include, inter alia, kinetic energy and rotational energy, energies of atom vibration, electron motion or atomic bonds. Internal energy is an extensive parameter that is defined by the state of the system, that is, its temperature, pressure, and the number of moles of its components. If we analyse a closed system in which the temperature and pressure parameters remain constant (T, V=const), the value of internal energy is the product of the number of moles and the molar internal energy. The latter, in turn, is the sum of the internal energies of individual components including the percentage content. The SI unit of energy is the joule (1 J); sometimes we also use values expressed in calories or electronvolts. When a gas that satisfy the ideal gas equation changes its volume, the internal energy of the system remains constant.
Enthalpy (H)
It is an important thermodynamic function that is defined as the sum of internal energy and the product of pressure and volume. This means that it depends on independent parameters which characterise the state of the system. It is an extensible quantity, i.e. it is additive. If parameters p and T of a closed system are constant, the enthalpy is the product of the number of moles of the substance and its molar enthalpy. It is important that, in a completed process, the change in enthalpy only depends on the initial state (Hinitial) and the final state (Hfinal), while the course of the process is irrelevant. A change in pressure does not result in a change in enthalpy of a system formed by a gas satisfying the ideal gas equation.
Energy between a system and its surroundings
Energy is capable of changing its forms, thus it can be transferred between a system and its surroundings as heat (Q) or work (W). Any work and heat added to a system raises its energy, so they must have positive values (Q>0, W>0). In contrast, all actions that reduce the system’s energy (work performed or heat emitted to the surroundings) are noted as negative values (Q<0, W<0). Heat effects are divided into two categories:
- endergonic, where heat is added to the system,
- exergonic, where the system releases heat energy to its surroundings.
Work performed by systems can also be divided; it may be:
- non-pressure-volume work – unrelated to the change in the system’s volume, or
- pressure-volume work – related to an external pressure acting on the system; this type of work changes the system’s volume.
The zeroth law of thermodynamics
The zeroth law of thermodynamics states that a body in thermodynamic equilibrium has the same temperature throughout. Therefore, the law assumes the existence of an empirical temperature that is equal in the system for all its parts which may exchange heat. This is since these parts tend towards thermal equilibrium which, besides chemical and mechanical equilibriums, is a precondition to obtain thermodynamic equilibrium.
The first law of thermodynamics
Also referred to as the law of conservation of energy, it is directly related to the changes in a system’s enthalpy or internal energy. It has two principal assumptions:
- The internal energy of an isolated system remains constant, regardless of the processes, if the system meets the following conditions:
U = const dU = 0 ΔU = 0
- The internal energy of closed non-isolated systems may change as a result of exchanging work and heat with the surroundings. That change is additive and equal to the sum of the energy added to or extracted from the system.
The most important consequence of the first law of thermodynamics is the fact that the system’s internal energy is regarded as a function of state that is independent of the process path.
The second law of thermodynamics
It is a principle that defines the direction of thermodynamic processes in the nature. It assumes that all phenomena proceed in the same irreversible direction. In an isolated system, there is a function of state called entropy (S), which does not decline over time. Entropy is the measure of a disordered system, and according to the second law of thermodynamics, an isolated system tends, by spontaneous processes, to states that are gradually less ordered. The simplest example to understand that law is the transfer of heat between two systems. The heat will always be transferred from hotter to colder objects, never the other way round.
The third law of thermodynamics
Also referred to as the Planck or Nernst–Planck postulate, it states that the entropy of a system in the state of full equilibrium (the state with the lowest energy) approaches zero as the temperature approaches absolute zero. We should note, however, that at the temperature of absolute zero all processes in the system stop, and also the specific heat and thermal expansion approach absolute zero. This means that the cooling of the system leads to the reduction of its entropy to very low values, but in practice it is impossible to achieve the temperature of absolute zero.